This web page was generated automatically, to access the article in its original form you can visit the link below:
https://www.nature.com/articles/s41556-024-01574-w
if you wish to remove this article from our website please get in touch with us
Optical redox imaging in epidermal stem cells over time
The mouse epidermis is perpetually renewed by stem cells situated in the basal layer (Fig. 1a(i),(ii)), all of which possess the ability to proliferate and restore the differentiated layers35. As of now, the relationship between the metabolic condition and cellular homeostasis in vivo in epithelial cells remains elusive. To explore this aspect, we modified our previously outlined two-photon microscopy deep-tissue visualization35,36,37 to include optical redox imaging22,23 and observe label-free fluorescence of the endogenous metabolites in the hairy (ear) and non-hairy (paw) skin of living mice (Fig. 1a(i)–(iii)). The intensities gathered for redox imaging at particular two-photon excitation wavelengths from intravital imaging are collected at wavelength ranges that correspond to NAD(P)H and FAD and can be represented post-imaging as a ratio NAD(P)H/ FAD (Fig. 1a and Supplementary Videos 1 and 2). To tackle the challenge posed by the undulating three-dimensional (3D) tissue in our evaluations of NAD(P)H and FAD fluorescence among hundreds of stem cells in the living organism, we isolated the fluorescence signal from the basal layer by using the distance from the interface of the epidermis and collagen (captured via the second harmonic signal) (Extended Data Fig. 1e,f).
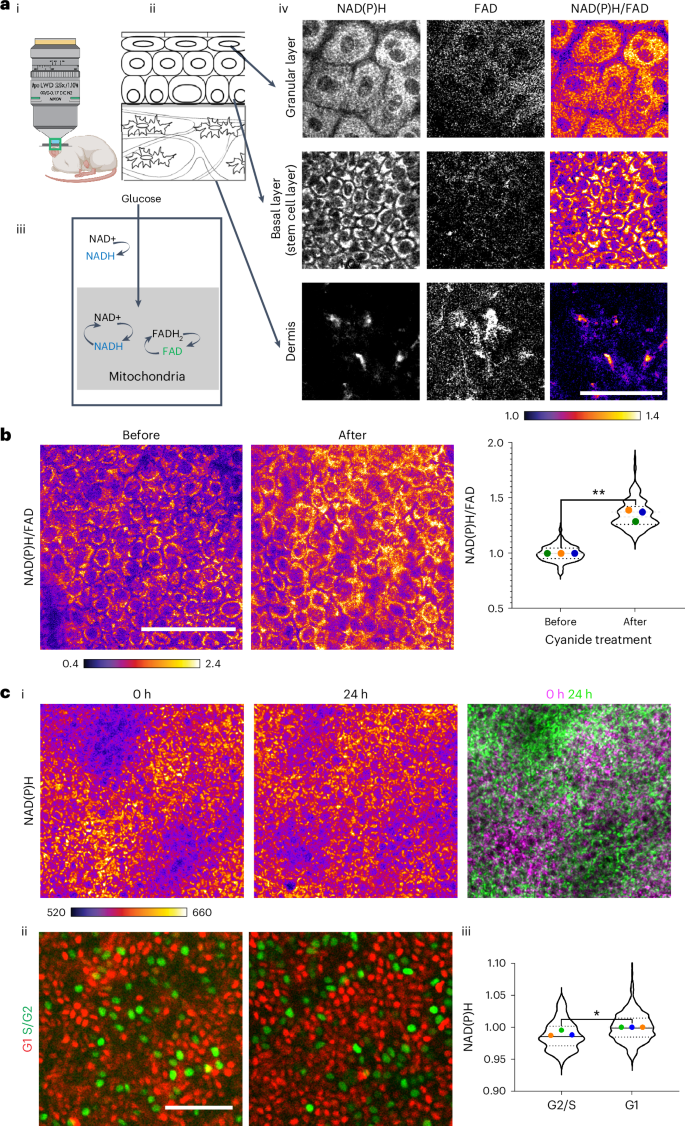
a, (i) Label-free imaging of NAD(P)H and FAD in the skin of living mice displays (ii) the dynamic metabolic signature of (iii) the stem cell compartment. Only the reduced and oxidized forms NADH, NADPH, and FAD are fluorescent and collectively they provide insights into the redox ratio of the cell. (iv) Two-photon images of various epidermal layers and cell types in skin epithelia capture the endogenous autofluorescence of the metabolic cofactors NAD(P)H and FAD. NAD(P)H/FAD levels in the epidermal stem cell compartment (basal layer) are elevated in comparison to cells in the dermis. The redox ratio (NAD(P)H/FAD) is depicted as an intensity plot (scale below). (iii) A schematic illustrating how alterations in glycolysis or mitochondrial metabolism rates impact the redox ratio. b, NAD(P)H/FAD ratios from the basal layer before and 5–10 min following the local injection of sodium cyanide. A rapid increase in NAD(P)H/FAD is plotted on the right. The coloured dots indicate average redox ratios per mouse from three mice, evaluated before and after the injection. **P = 0.0082 (paired t-test two-sided). Violin plot, n = 222 (before) and n = 187 (after) cells from three mice. c, (i) The basal layer upon reassessment after 24 h exhibits an altered distribution of NAD(P)H intensity patterns. The corresponding second harmonic signal patterns used to identify the same areas are shown in Extended Data Fig. 1b. (ii) Fucci (cell cycle reporter)-labeled stem cells. Green-labeled nuclei signify Geminin-containing nuclei (S/G2 cell cycle stage) and red-labeled nuclei signify Cdt1-containing nuclei (G1 stage). (iii) NAD(P)H intensities per cell assessed from G1 nuclei display an increase in mean intensity compared to S/G2 nuclei. Violin plot, n = 120 (S/G2) and n = 490 (G1) cells from three mice. The average value from each mouse is marked with a different colour. *P = 0.0063 (two-sided nested t-test). Scale bar, 50 µm. Panel a(i) designed with BioRender.com.
Source data
Considering the unique regenerative capabilities of epidermal stem cells, we inquired whether they possess a distinct metabolic signature when compared with other cell types in the dermis. Our findings indicated that cells in the epidermis have a high NAD(P)H intensity in relation to FAD (Fig. 1a(iv)). This contrasts with many cells in the dermis (Fig. 1a(iv) and Supplementary Videos 1 and 2). To verify if our technique accurately captured NAD(P)H and FAD intensities, we first treated 293T cells in culture with cyanide, which inhibits complex IV of the electron transport chain and results in the accumulation of NADH in the cells. We witnessed an immediate (2–5 min) rise in NAD(P)H intensities and NAD(P)H/FAD ratio (Extended Data Fig. 1a). We then proceeded to assess the redox ratios we ascertain in vivo by injecting sodium cyanide intradermally into living mice and promptly (5–10 min post injection) documenting the accumulation of NAD(P)H and heightened NAD(P)H/FAD from the same mice (Fig. 1b). This extreme and irreversible treatment signifies the upper limit of redox change that is physiologically feasible (28–37 % increase), underscoring the significance of sustaining redox ratios within a narrow range. Thus, in vivo, upon inhibiting mitochondrial oxidation, NADH accumulates leading to an increase in NAD(P)H/FAD, indicative of a more ‘reduced’ redox state.
Epidermal stem cells are consistently cycling through the cell cycle, which prompted us to question whether their metabolic signature is dynamic or stable. We revisited the same tissue area over hours to days to monitor its metabolic condition (described in Methods and Extended Data Fig. 1b). In the basal stem cell layer, large clusters (~25–50) of cells exhibited similar NAD(P)H intensities in a pattern that persisted over 2–3 h of imaging (Extended Data Fig. 1c). However, these clusters exhibited different relative NAD(P)H intensities when reassessed after 24 h (Fig. 1c(i) and Extended Data Fig. 1d). To comprehend how the cell cycle may affect NAD(P)H intensities, we employed a reporter that differentiates G1 cells (identified by mCherry-Ctd1) and S/G2 cells (identified by mVenus-Geminin-Fucci2 mice) (Fig. 1c(ii)). We noted a small yet significant difference between the average NAD(P)H intensities of S/G2 cells and G1 cells in the basal layer, with S/G2 cells exhibiting a relatively lower NAD(P)H intensity than G1 cells (Fig. 1c(iii)).
In summary, we demonstrate that epidermal stem cells possess a unique metabolic signature, with minor variations attributed to shifting cell cycle statuses in individual cells contributing to the range of redox values in homeostasis.
Redox alterations precede βcatGOF-induced anomalies
Wenext inquired how the redox states of epidermal stem cells alter with the expression of the oncogenic mutation βcatGOF (β-cateninflox(Ex3) (refs. 5,6)). Mice harboring βcatGOF; K14CreER underwent treatment with tamoxifen to activate the Cre recombinase within the basal stem cell layer (which produces keratin 14) and thus stimulate the expression of βcatGOF in a mosaic fashion. At day 5 following tamoxifen treatment, the basal layer was comprised of a single layer of nuclei, as seen in homeostasis, exhibiting heightened nuclear β-catenin localization compared to control cells (Fig. 2a). By day 15, unusual outgrowths featuring multiple layers of densely packed nuclei enriched with nuclear β-catenin had developed and extended into the dermis, mimicking hair follicle placodes (Fig. 2a, Extended Data Fig. 2a and Supplementary Video 5). To investigate changes in cell behavior, we stained for proliferation markers at the aforementioned time points and observed that there were no statistically significant modifications in the quantity of phospho-histone H3 (pH3)-marked nuclei at day 5 post-tamoxifen; however, a significant rise in pH3-labeled nuclei occurred at day 15 (Extended Data Fig. 2b,c). Therefore, at day 5 post-tamoxifen, despite β-catenin protein displaying increased nuclear (activated) localization compared to controls, we did not detect abnormalities in tissue structure nor quantitatively significant alterations in proliferation within the stem cell compartment of βcatGOF mosaic tissue (Fig. 2a, Extended Data Fig. 2 and Supplementary Videos 3–5).
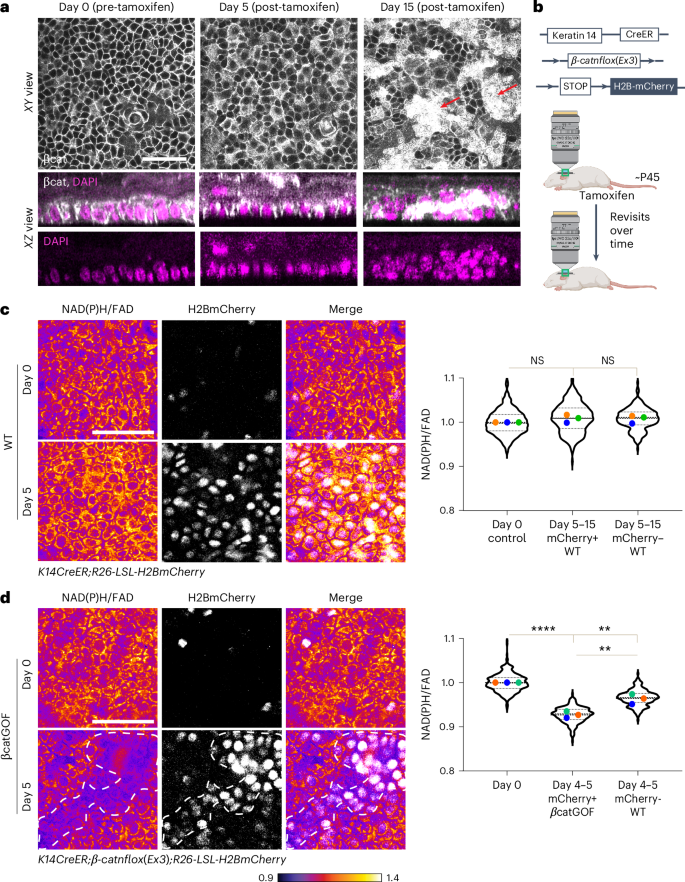
a, β-catenin immunofluorescence staining in the basal layer of the epidermis from K14CreER; βcatGOF mice 0, 5, and 15 days after tamoxifen-induced recombination and expression of βcatGOF. The xy (top) and xz (bottom) sections illustrate that the 3D architecture of the basal layer remains undisturbed at 5 days, in contrast to the multiple rows of nuclei observed in aberrant placodes at 15 days (red arrows; z-stack for day 0, 5, and 15 in Supplementary Videos 3–5; images in Extended Data Fig. 2b). b, Diagram illustrating that similar regions from the same animal were imaged both before and after tamoxifen-induced recombination and expression of βcatGOF and H2BmCherry (c,d). c, In control littermates (K14CreER; LSL-H2BmCherry), recombination following tamoxifen results in the expression of H2BmCherry (white nuclei). These recombined cells or their neighbors displayed no significant decrease in NAD(P)H/FAD ratio when imaged at 5, 9, or 15 days following tamoxifen administration. The average value from each mouse is marked with a different color for all graphs. Averages show no statistically significant differences (n = 3 each). Violin plot n (in order x axis) = 423, 250, and 221 cells from three mice. d, In βcatGOF mice (K14CreER; βcatGOF; LSL-H2BmCherry), mutant cells are identified by co-expression of nuclear H2BmCherry (white, outlined by white dashed line). Despite the absence of any other morphological irregularities, the NAD(P)H/FAD ratio of mutant cells (H2BmCherry-positive) dramatically declines after 5 days when compared to values at day 0. The neighboring WT cells (H2BmCherry-negative) also exhibit a decrease in redox ratio (NAD(P)H/FAD), albeit within a higher range than the mutant cells. Average redox P value derived from one-way analysis of variance (ANOVA); multiple comparisons; day 0 versus day 4–5 βcatGOF, ****P < 0.0001; day 0 versus day 4–5 WT, **P = 0.0027; day 4–5 βcatGOF versus day 4–5 WT, **P = 0.0032. n = 3 mice each. Violin plot n (in order x axis) = 336, 231, and 198 cells from three mice. Scale bar, 50 µm. NS, not significant. Panel b created with BioRender.com.
Source data
To explore whether metabolic alterations precede or follow these morphological abnormalities in the mutant mosaic epidermis, we assessed NAD(P)H and FAD levels in the stem cell layer starting at day 5 post-tamoxifen, prior to the emergence of abnormal phenotypes. To differentiate and track recombined versus unrecombined epidermal cells (for instance, βcatGOF versus WT in the mutant mosaic epidermis) over time in the adult mouse, we expressed Rosa26-CAG-LSL-H2B-mCherry (designated as LSL-H2BmCherry) in the basal stem cell compartment utilizing K14CreER (K14CreER; LSL-H2BmCherry) (Fig. 2b). Initially, we quantified the variance of redox values in control conditions including post-tamoxifen-induced recombination, expression of fluorescent alleles, and repeated imaging of the same epidermal region. We discovered that per-cell NAD(P)H/FAD is sustained at a stable average value (with a minor increase in range post-tamoxifen) in the homeostatic basal stem cells during revisits at 5, 9, or 15 days following tamoxifen treatment and the expression of fluorescent reporters (Fig. 2c).
To comprehend the metabolic shifts in basal cells within the context of the tissue and behavioral changes that occur following the induction of the βcatGOF mutation (Fig. 2a and Extended Data Fig. 2a), we utilized Cre-induced H2BmCherry and βcatGOF coexpression to pinpoint and monitor mutant βcatGOF cells over time (K14CreER; β-catnflox(Ex3); LSL-H2BmCherry). Approximately 80% of the stem cell layer was recombined and manifested H2BmCherry and βcatGOF. Five days post-induction, the βcatGOF mutant stem cells (H2BmCherry-positive) indicated a rapid decline in NAD(P)H/FAD ratio (Fig. 2d). This shift contrasts with what was noted in recombined WT animals and exhibits a much greater amplitude (7–10-fold higher). WT cells within the mosaic mutant tissue (H2BmCherry-negative) also demonstrated a reduction in NAD(P)H/FAD ratio, yet within a distinct and higher range than that of the mutant cells (Fig. 2d), implying that they react to changes in the metabolic status of adjacent cells. Consequently, the stem cell layer experiences a swift alteration in its redox state in response to the existence of βcatGOF cells before the onset of morphological and behavioral anomalies, making it one of the initial observable adaptations to the βcatGOF mutation.
As time progressed, the βcatGOF mutation induces modifications to stem cell proliferation and overall tissue architecture (Fig. 2a and Extended Data Fig. 2a). Therefore, we probed whether the early drop in redox ratio observed in these cells constitutes a permanent shift in metabolic state. By monitoring the NAD(P)H and FAD intensities in the same region of the basal stem cell layer over time (Extended Data Fig. 2d), we found that the cells expressing βcatGOF (H2BmCherry-positive) sustained a low NAD(P)H/FAD ratio range at 10 days post-tamoxifen, with minimal recovery from 5 days post-tamoxifen (Fig. 3a,b and Extended Data Fig. 2e). Conversely, the neighboring WT cells in the basal stem cell layer augmented their NAD(P)H/FAD ratio roughly 2.8-fold when juxtaposed with βcatGOF cells, aligning with homeostatic redox values (day 0) (Extended Data Fig. 2e). As a result, the redox differential between the βcatGOF cells and WT cells in the stem cell layer widened over time (Fig. 3c) due to the selective recovery of NAD(P)H/FAD ratios in WT cells.
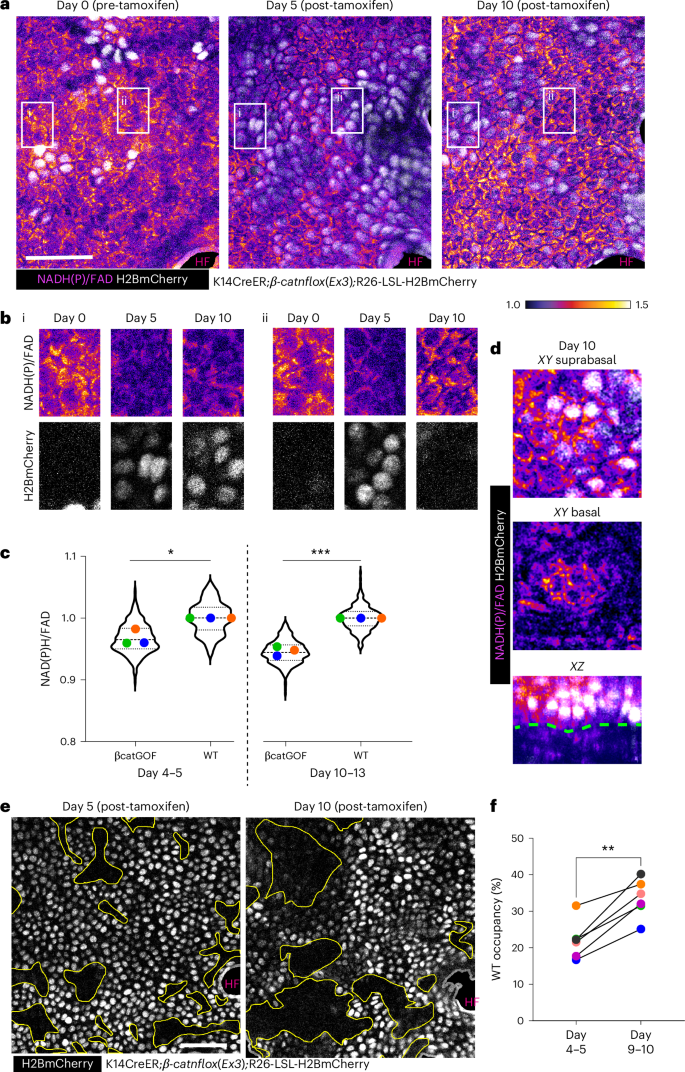
a, NAD(P)H/FAD intensities and H2BmCherry expression (white; signifies βcatGOF cells) from the same area (highlighted in yellow from larger regions depicted as Extended Data Fig. 3a) in K14CreER; βcatGOF; LSL-H2BmCherry mice analyzed 5 days and 10 days following tamoxifen administration. b, Insets highlighted in white from panel A, emphasizing regions over time where (i) βcatGOF cells at day 5 and day 10 exhibit marginal alteration in redox ratio and (ii) βcatGOF cells (H2BmCherry-positive) are supplanted by WT cells (H2BmCherry-negative), which display recovering redox ratios. c, Redox differential between mutant βcatGOF cells (H2BmCherry-positive) normalized to adjacent WT cells (H2BmCherry-negative) at days 4–6 and 9–11 post-tamoxifen indicates that the redox differential between βcatGOF cells and neighboring WT cells escalates between days 5 and 10 due to the preferential recovery of WT cells. Extended Data Fig. 2e presents the same data plotted relative to day 0. P value (two-sided t-test) derived from n = 3 mice each. *P = 0.012; ***P = 0.0002 Violin plot n (in order x axis) = 323, 198, 273 and 227 cells. d, At day 10 post-tamoxifen, the βcatGOF cells (H2BmCherry-positive) are displaced towards the suprabasal region, signifying differentiation (top), while WT cells occupy the basal layer (middle). Both layers can be observed in the xz section (bottom) with the blue outline representing the epidermal–dermal junction. e, The βcatGOF mosaic basal stem cell layer 5 days following tamoxifen contains neighboring patches of WT (H2BmCherry-negative; yellow outline) and mutant cells (H2BmCherry-positive), which were reanalyzed 10 days after tamoxifen; WT patches (regions highlighted in yellow) expanded between day 5 and day 10. f, Graph illustrates the area occupied by WT cells from the same 300 × 300 µm2 regions (n = 6) across three mice quantified, demonstrating an increase in coverage from day 5 to day 10. P = 0.0015 (paired t-test, two-sided). Scale bar, 50 µm.
Source data
Our prior research indicated that βcatGOF cells are removed from outgrowths over several months, aided by the presence and function of WT cells5. To enhance our understanding of the recovered metabolic signature of WT cells within the framework of their competitive advantage, we monitored the fates of WT and βcatGOF cells over time. We discovered that within the same skin region 5–10 days post-tamoxifen, the area occupied by WT cells, indicated by the lack of H2BmCherry, increased (Fig. 3e,f), while the H2BmCherry-positive mutant cells were progressively detected enriched in the suprabasal layer, with WT cells below (Fig. 3d), indicating that these mutant cells are expelled from the basal stem cell layer through differentiation. The mutant epidermis also exhibited a progressively more marked cell-competition phenotype when observed over an extended timeframe. At 1.5–2 months post-tamoxifen, a notable difference was evident in the occupancy of recombined cells in both the WT control littermates (LSL-H2BmCherry; K14CreER) and βcatGOF mosaic (βcatGOF; LSL-H2BmCherry; K14CreER) models. At this observation point, the recombined cells (H2BmCherry-positive) in WT control littermates still comprised about 80% of the basal stem cell layer, consistent with neutral drift, whereas in the βcatGOF mosaic epidermis, only roughly 25% of the basal stem cell region was occupied by H2BmCherry/βcatGOF-positive cells, indicative of a rapidly diminishing coverage of the mutant cells over time (Extended Data Figs. 3a and 9a). The remaining recombined H2BmCherry/βcatGOF-positive cells were predominantly grouped into 3D abnormal placodes extending into the dermis (Extended Data Fig. 3b and schematic shown in Fig. 6i) resembling hair follicles; this phenomenon also occurred in nonhairy βcatGOF mosaic skin (Extended Data Fig. 3c). Collectively, WT cells restore their redox status following the initial redox decline and ultimately outcompete βcatGOF cells to occupy greater area within the basal stem cell layer over time.
HrasG12V initially induces then reduces the redox differential
While βcatGOF mutant cells are outcompeted from the mouse epidermis by WT cells, other cell-competition models feature mutant cells that outcompete WT neighbors. This led us to question whether the metabolic variations resulting in altered redox and recovery patterns observed in the βcatGOF mosaic are unique to the mutation or indicative of broader cellular fitness during re-establishment of homeostasis. To explore this, we employed the HrasG12V (constitutively active Hras) mutation model, where the dynamic between WT and mutant cells is conversely different from that in the βcatGOF model: mutant HrasG12V cells outcompete WT cells in the basal stem cell layer5,7 (Extended Data Fig. 4a). Utilizing similar genetic backgrounds and experimental durations as the βcatGOF studies described previously, we examined how the NAD(P)H/FAD intensities in K14CreER; HrasG12V; LSL-H2BmCherry epidermal stem cells shifted upon tamoxifen-induced recombination and expression of HrasG12V. We noted an initial reduction in NAD(P)H/FAD ratios at 5 days post-tamoxifen (Fig. 4a–c and Extended Data Fig. 4b) in the basal stem cells when the same skin area was monitored over time (Extended Data Fig. 4a). By tracking the fluctuating redox across time points in which the recombined H2BmCherry/HrasG12V-positive cells proliferate (5–10 days post-induction), we investigated how the metabolic status of these cells evolved in light of their competitive advantage. Between 5–10 days post-tamoxifen, the NAD(P)H/FAD ratio of the H2BmCherry/HrasG12V-positive cells aligned with that of their adjacent WT cells (Fig. 4c). At 10 days post-tamoxifen, mutant cells and WT neighbors in the HrasG12V model altered their redox ratios (Fig. 4c and Extended Data Fig. 4b), thereby flattening the redox differential between mutant and WT cells. This contrasts with βcatGOF mutant cells, which intensified their redox differential with neighboring WT cells (Fig. 3c) and were ultimately surpassed by WT cells. Thus, through contrasting the HrasG12V and βcatGOF mutations with differing cell competition results, we uncover that both mutations initially decrease the redox ratio, yet in the HrasG12V mutant model, the redox differential between mutant and WT cells is diminished over time.
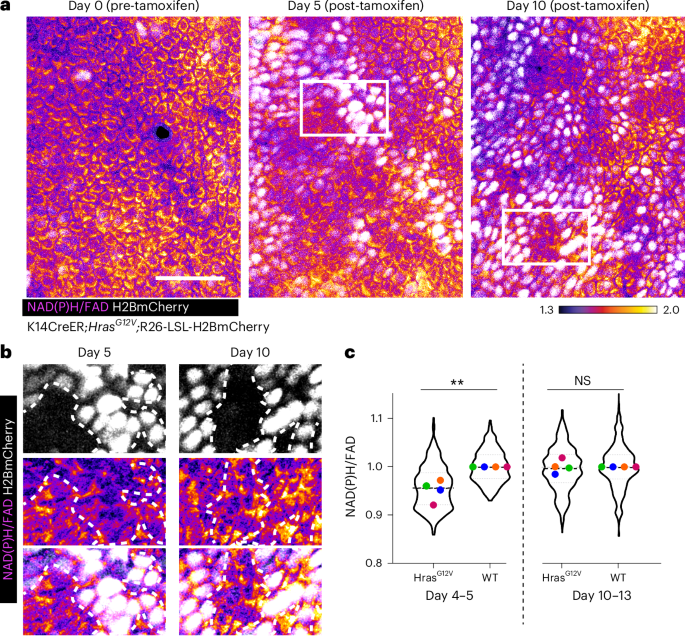
a,NAD(P)H/FAD intensities from identical epidermal areas (highlighted in yellow from broader regions illustrated in Extended Data Fig. 4a) examined at 0, 5–6, and 10–13 days subsequent to tamoxifen treatment and expression of HrasG12V and H2BmCherry (white) from K14CreER; HrasG12V; LSL-H2BmCherry mice. b, Insets from white delineated zones in a. Areas comprising HrasG12V and WT cells adjacent to one another indicate that on day 5 (left), HrasG12V (H2BmCherry-positive; demarcated by a white dotted boundary) cells exhibit a lower NAD(P)H/FAD intensity compared to WT cells (H2BmCherry-negative). By day 10 (right), HrasG12V (H2BmCherry-positive) cells display an increase and restore their NAD(P)H/FAD intensities, now comparable to neighboring WT cells (H2BmCherry-negative). c, Evaluation of NAD(P)H/FAD intensities from HrasG12V (H2BmCherry-positive) cells normalized against their WT neighbors (H2BmCherry-negative) on day 4–5 (left) and day 10–13 (right) to illustrate that the redox differential observed at day 4–5 is leveled by day 10–13. This contrasts with βcatGOF epidermis, where the redox differential between mutant and WT amplifies (Fig. 3c). Each mouse’s average value is represented with a distinct color. Average redox P value (two-sided t-test) from four mice, **P = 0.0044 for day 4–5 HrasG12V versus WT; not significantly (NS) different for day 10–13 HrasG12V versus WT. Violin plot n (in order x axis) = 425, 228, 560, and 226 cells from four mice. Extended Data Fig. 4b presents the same data plotted concerning day 0. Scale bar, 50 µm.
Source data
Alterations in glucose catabolism underpin redox variations
NAD(P)H and FAD ratios indicate the relative equilibrium of reduced compared to oxidized metabolites within a cell, which in turn is influenced by the relative rates of glycolytic versus mitochondrial activity. To ascertain the mechanistic and biochemical foundations of the variations in NAD(P)H to FAD intensities observed through live imaging in the mutant models outlined thus far, we sought to determine how the glucose catabolic rates were altered in those models. We noticed that the trend of redox change in βcatGOF and HrasG12V models was aligned with drug treatment (dichloroacetic acid; DCA) that redirected more glucose into the tricarboxylic acid (TCA) cycle and subsequent oxidation (Extended Data Fig. 4c,d). Initially, we applied untargeted metabolomics and pathway enrichment analysis to evaluate global metabolic shifts, from which glycolysis and the TCA cycle emerged as some of the most affected metabolic pathways in βcatGOF and HrasG12V when juxtaposed with WT littermates (Extended Data Fig. 5 and Supplementary Table 1). The metabolomics dataset also revealed a decrease in levels of pentose phosphate pathway components and a buildup of several amino acids. To further investigate how specific stages of glucose metabolism likely influence the redox state of the mutant cells, we conducted targeted LC–MS/MS-based stable isotope tracer analysis after infusing 13C isotope-labeled glucose into P45–P62 mice, 6 days post high doses of tamoxifen to induce expression of βcatGOF or HrasG12V in the majority, if not all, epidermal cells. Epidermal tissue isolated from the mice infused with 13C6 glucose (Methods) was then utilized to evaluate label retention at diverse stages of glycolytic and oxidative metabolism to determine relative fluxes through various steps (Fig. 5a)34. We discovered that VPDH/VCS (the ratio of pyruvate dehydrogenase flux to citrate synthase flux), the portion of the TCA cycle powered by glucose34,38,39, indicated by 4,5-13C2glutamate/13C3-alanine (Extended Data Fig. 6b) and the fractional contribution of glucose to acetyl-CoA, 13C2-acetyl-CoA/13C3-alanine (Fig. 5b) were elevated in both βcatGOF and HrasG12V epidermis (acetyl-CoA merges inputs from glucose and fatty acid metabolism). Hence, the TCA cycle was predominantly driven by glucose in both mutant tissues. Given that the TCA cycle and mitochondrial oxidative phosphorylation are closely linked, it signifies that mutants enhance glucose consumption for mitochondrial oxidation. The upsurge in 13C-labeled CO2 in relation to glucose was also noted in both mutant tissues (Fig. 5c), directly signifying that the flow of glucose through the TCA cycle and subsequent oxidation was intensified by the mutations. Notably, this increased glucose utilization for mitochondrial oxidation aligns with the reduction in NAD(P)H to FAD ratio (a more oxidized redox state) that we observed through live imaging (Figs. 2c,d and 4a–c), providing a direct biochemical connection.
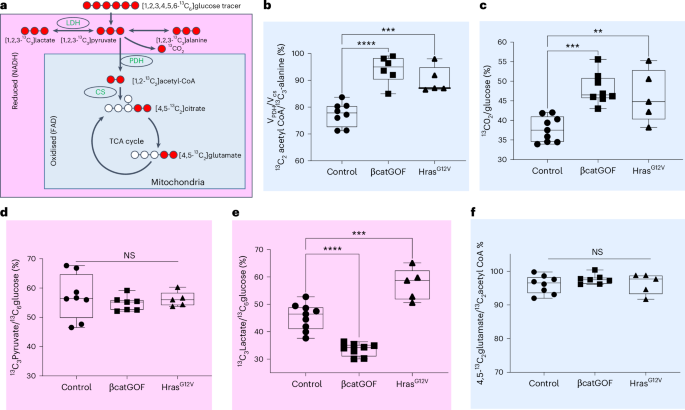
a, Diagram illustrating the labelling of carbon (red) in downstream metabolites upon infusion of 13C6 glucose into mice. The outputs linked to reduced NADH in the cytosol are marked in pink, while those connected to mitochondrial metabolism are marked in blue. b, The ratio of 13C2-acetyl-coA/13C3-alanine, also referred to as VPDH/VCS, denotes the percentage of the TCA cycle driven by glucose. When compared to WT controls, this ratio elevates to nearly 100%, indicating that the βcatGOF and HrasG12V are utilizing glucose to its fullest extent for TCA cycle and subsequent glucose oxidation. n = 8 (control), 6 (βcatGOF) and 5 (HrasG12V) mice, P values, ****P < 0.0001; ***P = 0.0005. c, Another indication of TCA flux, 13CO2 normalized to 13C6 glucose, also rises in both mutants. n = 9 (control), 8 (βcatGOF) and 5 (HrasG12V) mice, P value, ***P = 0.0003; **P = 0.0058. d, In contrast, labelled pyruvate normalized to labelled glucose shows no change in either mutant. n = 8 (control), 7 (βcatGOF) and 5 (HrasG12V) mice. e, Labelled lactate relative to glucose, decreases in βcatGOF, while in the HrasG12V epidermis, the 13C3 labelled lactate to 13C6 glucose ratio increases. Consequently, the mutant HrasG12V epidermis generates more lactate from pyruvate compared to the βcatGOF tissue, in which this conversion is lower than WT controls. n = 9 (control), 8 (βcatGOF) and 5 (HrasG12V) mice, P values, ****P < 0.0001;***P = 0.0002. f, 13C2-labelled glutamate to acetyl-CoA measures the dilution of the label due to glutamine entry into the TCA cycle. n = 8 (control), 7 (βcatGOF) and 5 (HrasG12V) mice. The nearly 100% ratios suggest that glutamine entry is minimal. This is not significantly different in either mutant epidermis when compared with WT epidermis. All graphs employ a one-way ANOVA (multiple comparisons relative to control). Every point representsepidermis (ear) from a single mouse. All box plots illustrate all points with whiskers extending from minimum to maximum, accompanied by a line indicating the median.
Source data
To ascertain whether glycolysis was altered, we concentrated on pyruvate and lactate labeling originating from glucose, which is anticipated to be elevated in oncogenic mutations, in alignment with the Warburg effect19. At the concluding phase or readout of glycolysis, particularly the transfer of 13C label from glucose to pyruvate, there was no corresponding transformation in the labeling of pyruvate sourced from glucose in either mutant epidermis (Fig. 5d). However, upon examining the interplay between pyruvate and lactate, the two mutations exhibited distinct influences: cells within the HrasG12V epidermis generated more lactate from glucose, whereas cells in the βcatGOF epidermis produced less (Fig. 5e). Collectively, these findings suggest a specific and differential modulation of the pyruvate to lactate conversion in both mutants when compared to control epidermis (Extended Data Fig. 6c). Given that NADH is produced at earlier glycolytic stages before pyruvate, these notable changes in the pyruvate to lactate conversion are consistent with the more oxidized redox ratio seen in both mutants. The 13C-labeled glutamate derived from 13C-acetyl-CoA (m + 2 C4C5-glutamate/m + 2-acetyl-CoA) showed no differences in the two mutant models (contrary to the ratio of labeled glutamate to pyruvate), indicating a minimal and comparable contribution of glutamine to the TCA cycle and mitochondrial oxidation (dilution of glutamate by unlabeled glutamine entry) between the WT and the two mutant models (Fig. 5f and Extended Data Fig. 6e). There is also no dilution of the 13C at the later stage of m + 2 malate compared to glutamate (2,3-13C2 malate/4,5-13C2-glutamate; Extended Data Fig. 6f). For these investigations, 2,3-13C2 malate was treated as an integrated average of 1,2-13C2 and 3,4-13C2 malate (both of which cannot be measured by MS), emphasizing the fraction of anaplerosis producing C3C4 malate in comparison to C1C2 malate. Thus, the primary alterations in glucose oxidation occur at the level of glucose entry into the TCA cycle, with no evidence of modified anaplerosis from other substrates (Fig. 5f and Extended Data Fig. 6d–f).
Metformin reverses mutant characteristics and cellular competition
The decline in NAD(P)H/FAD levels, the rise in VPDH/VCS, and the increase in 13CO2/13C6-glucose all signify a greater fractional contribution of glucose to mitochondrial oxidation in both βcatGOF and HrasG12V epidermis. To examine whether these modifications are merely correlated with the oncogenic mutant phenotype or are causative, we administered metformin to mice, a mild inhibitor of mitochondrial function40,41,42,43, previously characterized in oncogenic models42,44 and acknowledged for altering cytosolic redox to a more reduced state45,46. It is important to note that various mechanisms of action of metformin have been documented, and its systemic effects are likely not confined to mild inhibition of mitochondrial activity; however, since the majority of evidence points to its role in diminishing mitochondrial oxidation47 within a physiologically relevant range48, we chose it for further examination of phenotype and flux.
Mutant βcatGOF mice were given daily doses of metformin48 in drinking water for ongoing exposure to the drug. Initially, we ascertained that homeostatic structure and function of skin epidermis, including the morphology of various epidermal layers, proliferation rates, and differentiation patterns (Extended Data Fig. 7a–d) of the epidermal stem cell compartment, remained unaffected in WT littermates. Next, we assessed the impact of metformin on glucose metabolism in the epidermis of WT and mutant βcatGOF and HrasG12V mice post infusion of 13C6 glucose. Consistent with a reduction in mitochondrial oxidation, 13C2-acetyl-CoA/13C3-alanine and 13CO2/13C6 glucose decreased in the epidermis of both WT and mutant mice (Extended Data Fig. 8a,b). Notably, the reduction in glucose contribution to the downstream TCA and oxidation was more pronounced in both βcatGOF and HrasG12V mutants, eliminating the differences between the WT and mutant epidermis; highlighting their reliance on optimal mitochondrial function for metabolic rewiring by the mutant cells. Aligning with the decreased pyruvate (derived from 13C6-glucose) directed to the TCA cycle and glucose oxidation, we observed an increase in 13C3-lactate/ 13C6-glucose and 13C3-lactate/13C3-pyruvate following metformin treatment, significantly in WT and βcatGOF mutants (Extended Data Fig. 8c,d). The Hras G12V mutant that exhibited elevated 13C3-lactate/ 13C6-glucose even before treatment, maintained similar levels, suggesting that Hras mutant cells are already at an optimal rate of pyruvate to lactate exchange. Metformin also induces a mild dilution of 13C-labeled glutamate derived from 13C-acetyl-CoA (within 85–90%), indicative of some glutamine anaplerosis (Extended Data Fig. 8e) as reported earlier49. Thus, metformin decreases glucose oxidation and normalizes the metabolic differences between WT and mutant βcatGOF and HrasG12V mutant epidermis.
To evaluate the effects of metformin on the redox ratio following mutation induction, we assessed NAD(P)H/FAD levels at 5 days, revealing that metformin-treated βcatGOF animals exhibited no significant change in redox (Fig. 6a,b and Extended Data Fig. 7e,f) between WT and mutant cells at day 4–5 post-mutation induction, reflecting the normalization of the glucose metabolic differences between WT and mutant βcatGOF after metformin treatment (Extended Data Fig. 8). However, the variability in per-cell redox upon metformin treatment was greater than in untreated mice, highlighting the variations in drug penetration. To determine the impact of this treatment on a tissue level, we monitored the treated and untreated mutant mice up to 3 weeks after treatment, a duration during which the βcatGOF mosaic mutant animals develop epidermal outgrowths that distort the collagen and penetrate into the dermis (Fig. 6c,d and Supplementary Video 6). Notably, in βcatGOF mutant animals that received metformin, there was a significant reduction in both the number and size.of these mutant-induced abnormal epidermal growths when contrasted with untreated mutant mice (Fig. 6c,d,g and Supplementary Videos 6 and 7). To delve deeper into the cellular manifestation of the cell-competition phenotype, we monitored H2BmCherry-positive (representing mutant cells) versus H2BmCherry-negative (WT cells) areas at 2–2.5 months following the induction of βcatGOF. In mutant mice undergoing metformin treatment, the H2BmCherry-positive cells are predominantly not eradicated and are astonishingly still present in most of the epidermis (~60%) (Fig. 6f,h) unlike the situation observed in mutant mice without treatment (~20%; Fig. 6e,h). The ‘loser’ phenotype of βcatGOF is visually pronounced in the revisited mice at this stage, where extensive skin regions devoid of H2BmCherry-positive mutant cells are prominent, previously filled with mutant cells (Fig. 6h and Extended Data Fig. 9a). Conversely, the epidermis of metformin-treated mutant βcatGOF (Fig. 6h and Extended Data Fig. 9b) mice remains occupied by H2BmCherry-positive mutant cells, closely resembling control animals (littermates where only H2BmCherry was recombined and revisited; ~80% H2BmCherry occupancy; Extended Data Figs. 3a and 9c).
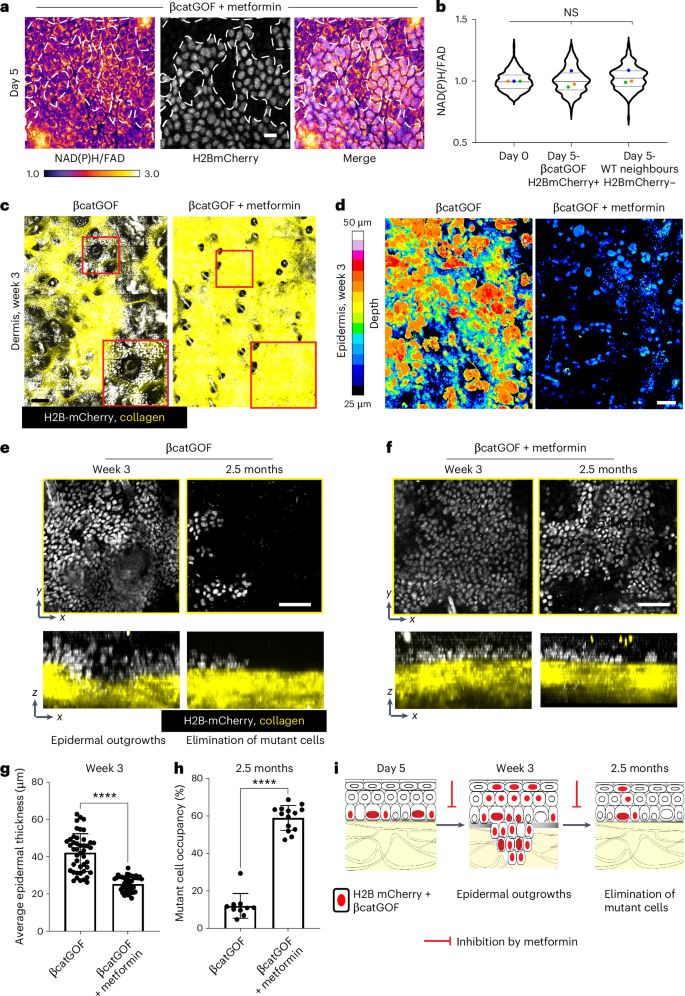
a,b, In βcatGOF mice (K14CreER; βcatGOF; LSL-H2BmCherry), subjected to metformin, 5 days post-induction of mutation in a mosaic fashion, there is no observable difference in the redox ratio in both recombined H2BmCherry-positive (white nuclei) βcatGOF mutant cells and their WT neighbors (H2B-mCherry-negative), compared to day 0 (prior to recombination; images in Extended Data Fig. 7e), presented in b. One-way ANOVA of means along with nested multiple comparisons indicates no significant variations. Violin plot: n (in order of x axis) = 326, 228, and 201 cells from three mice. Extended Data Fig. 7f visualizes the same data comparing mutant and WT neighbors. Scale bar, 10 µm. c, Maximum projection of z-stacks from epidermis and dermis featuring epidermal H2BmCherry-positive βcatGOF mutant cells (white) and collagen via second harmonic imaging (SHG; yellow) in vivo (magnified in red insets). In βcatGOF mutant animals, epidermal outgrowths are apparent at week 3 post-induction (day 19–21) protruding into the dermis (video through the z-section also in Supplementary Video 6). After metformin treatment, these epidermal protrusions become invisible in the maximum projections through the dermis (see i for schematic). d, The depth of epidermal protrusion from the top of the cornified layer from fixed epidermal preparations of βcatGOF mice compared to those administered metformin. The depth is color-coded as per the lookup table (LUT) to the left and quantified in graph g. While the βcatGOF epidermis shows greater (yellow/red) epidermal outgrowths at week 3, such outgrowths are either non-existent or markedly diminished in size in the metformin-treated mice. Scale bar, 100 µm (c,d). e, Upon revisiting the βcatGOF subjects after 2.5 months, H2B-mCherry-positive βcatGOF cells (white nuclei) are largely absent from zones they previously occupied at week 3. f, In contrast, βcatGOF subjects treated with metformin, upon revisitation at 2 months, still retain a majority of H2B-mCherry-positive βcatGOF cells. Both e and f depict zoomed-in regions cropped from larger ~1.8 mm2 areas in Extended Data Fig. 9a,b where disparities in mutant cell occupancy can also be observed. g, Thickness measurement from the top of the epidermis to the bottom plotted (images in D) demonstrating the reduction in the depth of outgrowths in βcatGOF mutant epidermis upon metformin treatment. n, average thickness from nine regions per mouse ranging from 400–500 µm2 across five mice for both mutant and metformin-treated. P value ****P<0.0001 (two-sided t-test). h, Percentage of area occupied by mutant (H2BmCherry-positive cells) quantified (images in e,f; Extended Data Fig. 9a,b) n = 3-4 regions per mice (size 700–1,200 µm2) from three mice each. P value ****P < 0.0001 (two-sided t-test) Data are articulated as mean ± s.d. (g,h). Scale bar, 50 µm (e,f). i Schematic illustrating the temporal sequence of events in the βcatGOF mutant epidermis where mutant cells are confined within epidermal outgrowths at weeks 2–3 (leading to WT cell proliferation in the basal layer; Fig. 3e,f), succeeded by extensive removal of mutant cells by 2–2.5 months.
Source data
Consequently, while the metformin-treated mice do not exhibit any morphological irregularities or epidermal outgrowths at 3 weeks, mutant cells are also not efficiently eliminated from the skin and consequently, their cell-competition results are reversed. Notably, the epidermis of βcatGOF treated with metformin remains hyperproliferative (Extended Data Fig. 10a,b) and the prevention of morphological alterations and cell-competition outcomes cannot simply be attributed to proliferation changes. Moreover, keratin 10 (K10; differentiation marker) staining of metformin-treated βcatGOF mosaic epidermis reveals a distribution of K10+ cells akin to wild-type50, contrasting with the extensive ectopic clusters of K10+ cells adjacent to pH3+ cells surrounding outgrowths in untreated βcatGOF mosaic epidermis (Extended Data Fig. 10c,d).
To further investigate whether metabolic reprogramming of cellular redox and glucose oxidation influences the phenotype of mutant HrasG12V mice, we examined the effects of metformin treatment on the HrasG12V mutant epidermis. The HrasG12V cells in animals treated with metformin also do not experience a decrease in NAD(P)H/FAD (Fig. 7a,b and Extended Data Fig. 7g,h), unlike our previous findings in mutant animals (Fig. 4c). The distinctive phenotype of HrasG12V mutant epidermis (hyper-thickening at day 5, augmented basal layer (stem cell layer) cell density at day 10, and increased proliferation) is all diminished when animals receive metformin treatment (Fig. 7c–h). Therefore, the phenotypes downstream of HrasG12V were curtailed, leading to a loss of proliferative superiority and mutant characteristics by the mutant cells.
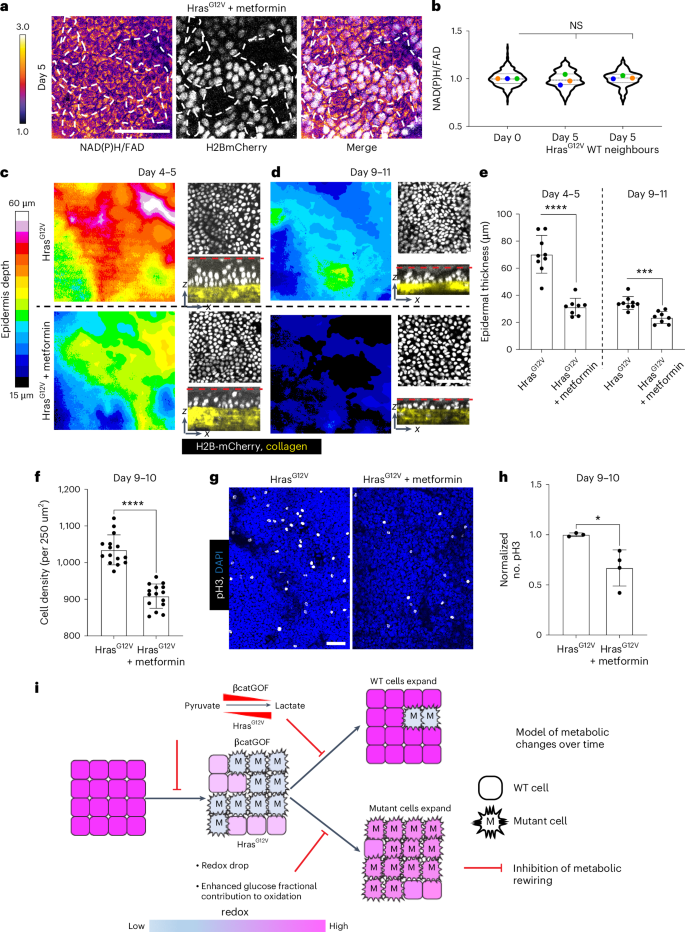
a,b, In HrasG12V mice (K14CreER; HrasG12V; LSL-H2BmCherry), administered metformin, 5 days post-induction of mutation in a mosaic manner, there is no alteration in the redox ratio in both recombined
H2BmCherry-positive (white nuclei); HrasG12V mutant cells and WT neighbors (H2B-mCherry-negative), when assessed against day 0 (prior to recombination; visuals in Extended Data Fig. 7g); illustrated in b. A one-way ANOVA of means, nested with multiple comparisons, indicates no noteworthy alterations. Violin plot, n (in sequence x axis) = 307,196 and 136 cells from three mice. Extended Data Fig. 7h illustrates the same data with mutant and WT neighbors compared against one another. c,d, The rise in epidermal thickness (color-coded in the LUT bar on the left) in HrasG12V mutant epidermis is curtailed upon treatment with metformin both 4–5 days (thickest) and 9–11 days following mutation induction. xz sections depicting epidermal nuclei between the cornified layer (red dotted line) and collagen imaged through the second harmonic signal in yellow highlight hyper-thickening in HrasG12V mutant mice relative to metformin-treated mutant mice, contrasting top (HrasG12V) with bottom (HrasG12V + metformin). The hyper-thickening is predominantly resolved by day 9–11 in HrasG12V mice. e, A graph quantifying epidermal thickness depicted in images in c,d. The identical regions were reevaluated between days 4–5 and day 9–11. n = 3 regions of interest, each measuring 300 µm2 from three mice for mutant and metformin-treated. P value (two-sided t-test) ****P < 0.0001; ***P = 0.0002. f, Cell density within the basal stem cell layer quantified at days 9–10 from epidermal preparations shows metformin treatment suppressing the elevated density in HrasG12V mice; also observed in the xy panels depicted in d; contrasting top (HrasG12V) with bottom (HrasG12V + metformin), n = five 250-µm2 regions from three mice each. P value (two-sided t-test) ****P < 0.0001. g,h, pH3-positive dividing cells in HrasG12V mice and HrasG12V mice administered with metformin demonstrate a decline in the proliferation rate quantified in h. n = 3, 4 mice each for Hras and Hras + metformin (normalized from 900–1,200 µm2 regions). P value (two-sided t-test) *P = 0.026. Results are presented as mean ± s.d. (e,f,h). Scale bar, 50 µm (a–g). i, A model illustrating how metabolic reconfiguration drives tissue phenotypes yielding opposing cell-competition outcomes. Following the induction of βcatGOF and HrasG12V mutations, there is a rapid decrease in NAD(P)H/FAD of epidermal stem cells in both mutant and WT cells at initial time points, indicating a more-oxidized redox ratio. The mutant epidermis also amplifies the relative flux of glucose through the TCA cycle and mitochondrial oxidation, consistent with a more-oxidized cellular redox state. Revisits over time reveal that in the βcatGOF mutant model, the redox differential between victorious WT cells neighboring the mutant cells persist, while in the HrasG12V mutant model, the redox differential is solely transient and diminishes with time. Concurrently, βcatGOF mutant cells are outcompeted by WT cells, and HrasG12V cells proliferate in the basal stem cell layer of the epidermis. Upon moderate suppression of mitochondrial oxidation by metformin, the redox decline in the mutant mosaic epidermis is inhibited, and downstream tissue phenotypes are also impeded, resulting in the inhibition of cell-competition outcomes, specifically the eradication of βcatGOF cells and the proliferative advantage of HrasG12V cells.
Source data
In sum, our experiments showcasing the redox decline in mutant epidermis, modifications in mitochondrial substrate utilization, and metformin-mediated suppression of metabolic reconfiguration, illustrate that obstructing the mutant-driven alterations in cytosolic redox and mitochondrial glucose oxidation within the epidermal stem cell compartment inhibits downstream βcatGOF and HrasG12V cellular and tissue transformations that foster their contrasting cell-competition phenotypes. These findings demonstrate that initiating metabolic alterations subsequent to mutations in βcatGOF and HrasG12V is the mechanism by which these mutations exert their influence on the tissue.
This page was created programmatically; to view the article in its original context, you can follow the link below:
https://www.nature.com/articles/s41556-024-01574-w
and if you wish to remove this article from our site, please contact us.