This page was generated automatically; to read the article in its initial location, please follow the link below:
https://today.uconn.edu/2025/01/black-hole-archaeology-understanding-how-black-holes-gained-their-mass/
and if you wish to have this article removed from our site, kindly get in touch with us.
Logan Fries, a doctoral student in Physics at the University of Connecticut, has shared intriguing discoveries regarding adjacent black holes at a press event on Tuesday, Jan. 14 during the 245th gathering of the American Astronomical Society in National Harbor, Maryland. The title of Fries’ presentation, “Black Hole Archaeology: Mapping the Growth History of Black Holes Across Cosmic Time,” outlines his research on measuring the spin of black holes, demonstrating that the majority have amassed mass through processes driven by accretion rather than through merger incidents.
“We’ve initiated a very ambitious new project exploring spinning black holes from the universe as it exists today and as far back as just over seven billion years ago,” Fries mentions, who is part of the research team of UConn Department of Physics Associate Professor Jonathan Trump.
According to Fries, this topic has not been extensively studied until now, mainly due to the requirement for precise measurements of black hole mass. However, thanks to the Sloan Digital Sky Survey Reverberation Mapping project (SDSS-RM)—an international collaboration in which Fries and Trump participate—these comprehensive mass evaluations have already been conducted for hundreds of black holes.
“I have come across research articles that discuss black hole spin from a theoretical perspective, focusing on black hole mergers, and I was interested in whether spin could be measured through observation,” states Fries.
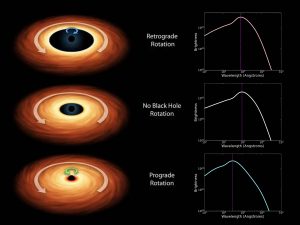
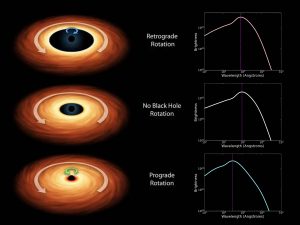
At the core of a black hole, there exists an accretion disk where matter accumulates as it falls into the black hole. Measurements of mass and accretion rate can provide valuable information regarding the history of the black hole, yet the spin of black holes remains the most difficult aspect to measure. Fries elucidates that the method used for this research is based on measurements taken from a region of the accretion disk known as the innermost stable circular orbit, or ISCO region.
“This is the area where material like gas is circulating, being accreted, and eventually will be consumed by the black hole,” he clarifies. “I refer to this as ‘black hole archaeology’ because we aim to comprehend how the mass of that black hole has developed over time. By analyzing the black hole’s spin, you are essentially examining the fossil record. For example, if a black hole has only been accumulating material in an organized disk since its formation, it will experience a rapid increase in spin. However, if two galaxies come together, their random orientations will cause the resultant merged black hole to have a low spin.”
Fries notes that there are black holes situated at the center of every galaxy, and the dominant theory on how galaxies evolve over time involves galaxy mergers. Thus, we should observe low-spinning black holes in the nearby universe.
“What is fascinating about black holes is that they appear so exotic, yet if one simply understands the mass and the spin, one can comprehensively describe that black hole using just these two values.”
Ultraviolet optical measurements of the supermassive black holes were captured utilizing the Galaxy Evolution Explorer (GALEX) and the Panoramic Survey Telescope and Rapid Response System (Pan-STARRS). One challenging aspect of this research is that black holes can fluctuate between measurements since conditions can shift rapidly. Therefore, this type of study demands observations and measurements taken promptly over a short time frame, lasting around 10 days to ensure that they are contemporaneous, says Fries.
“This approach allows us to assume that these measurements accurately represent the black hole during the same time period, before it has the opportunity to become brighter or dimmer.”
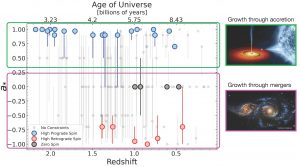
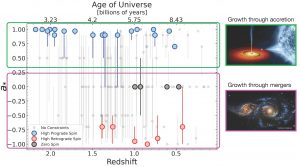
The researchers subsequently devised an accretion disk model that incorporates mass, accretion rate, and spin as input factors to produce “spectral energy distributions” or SEDs, which are models that represent how luminous the black hole appears at specific light wavelengths. They then apply multi-parameter nonlinear regression to align the UV and optical sections of the SED with the data to restrict the spin of the black hole.
Fries mentions that the key outcome from this study is the discovery of a significant number of rapidly spinning black holes within the nearby universe:
“This defies our expectations as a field regarding the development of galaxies and the growth of black holes through galaxy mergers, which should lead to a reduction in black hole spin. Gaining a clearer understanding of how black holes have originated and expanded throughout cosmic history offers important insights on galaxy evolution, broader accretion physics, and cosmology. This is particularly crucial as the James Webb Space Telescope has been identifying supermassive black holes that are larger than we would typically anticipate at this point in the universe.”
Fries has consistently held an interest in physics and astronomy, arriving at UConn in Fall 2019 to pursue his Ph.D. He anticipates completing his degree in Spring 2025.
This research has been supported by NSF CAREER-1945546, AST-2009539, and AST-2108668.
This page was generated programmatically; to view the article in its initial location, you may visit the link below:
https://today.uconn.edu/2025/01/black-hole-archaeology-understanding-how-black-holes-gained-their-mass/
and if you wish to remove this article from our site, please get in touch